Getting to the final lap of the magnetic racetrack
In the future, magnetic hard drives look set to be replaced by magnetic racetrack memory devices, and our researchers are seeking to understand the physics of this racetrack magic.
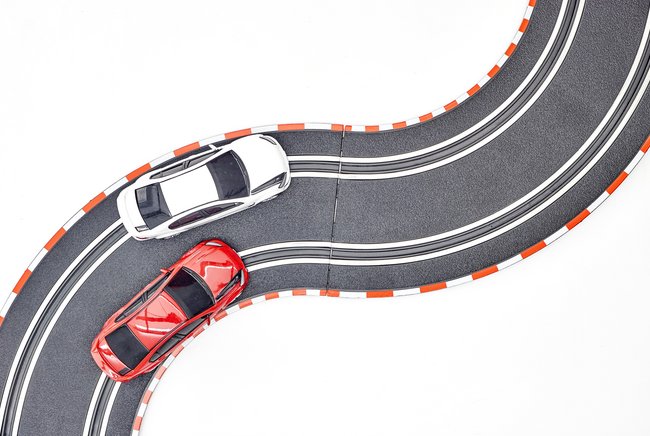
Our hard disks currently are far from sustainable: they wear down, are expensive, and are not very energy efficient. A potential solution is a so-called magnetic racetrack memory where whirling data-storing magnetic structures whizz around a nano-sized racetrack. There are no moving parts, it’s more energy efficient, and cheaper. But before we buy our tickets for the magnetic racetrack, we need a better understanding of how racing magnetic structures interact with each other. Mariëlle Meijer and her TU/e colleagues are very much attracted to the challenge.
Magnets do a lot more than just decorate our home appliances with holiday ornaments, because for years, magnetic materials have stored our valuable data in hard drives.
But why use magnetic materials to store data? Well, at the smallest scale, these materials are made up of domains of magnetic spins. These spins lead binary lives in which they can be pointing either up or down. Computer data, as a series of bits or 1s and 0s, can then be mapped to these up and down spins quite easily.
In magnetic-based hard disks, data stored in millions of spins is accessed using a read/write head, but this leads to problems which Mariëlle Meijer, PhD candidate in the department of Applied Physics at Eindhoven University of Technology, is quick to highlight.
“Read/write heads need to move across a disk. This wears materials and uses energy inefficiently,” says Meijer. “It would be better if the head were stationary, and if the data-storing magnetic domains were carried past the read/write head by an electric current.”
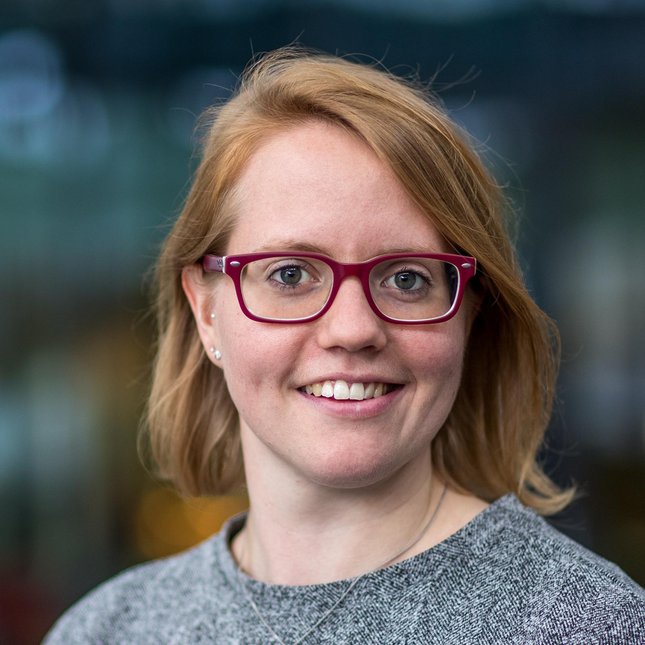
Let’s all go to the racetrack
As part of her research, Meijer is seeking to unravel the mysteries of so-called magnetic racetrack memory, a technology that has gained considerable attention over the past decade. Since 2017, Meijer has worked in the Physics of Nanostructures group in close collaboration with Reinoud Lavrijsen, as well as former PhD researcher Juriaan Lucassen.
“Magnetic domains with spins pointing up or down is the simplest way to think of data in magnetic materials. But other profiles for data storage are possible, and can be found on the walls between the domains. These are known as magnetic domain walls,” says Meijer.
In racetrack memory, when a current passes through a material that is home to magnetic domain walls, the magnetic domain walls are moved through the material. The flow of domain walls then races by the read/write head of the memory device. “These structures are whirling structures, which makes the racetrack a spintronic device,” notes Meijer.
Importance of chirality
In spintronic devices, chirality is important. But what does that mean? Hold your hands up in front of you. You’ll notice that you can’t map them exactly on top of each other, even if you rotate or move them relative to each other. This is chirality or handedness.
Magnetic structures, like magnetic domain walls, also display chirality in terms of how they are rotating, whether that is clockwise or anti-clockwise. That’s where chiral magnetic domain walls come from, and on the racetrack, chirality is a key factor in terms of how magnetic structures interact with each other.
Meijer’s work is one step back from trying to build functional magnetic racetrack memory. She’s interested in studying the interactions between these magnetic structures and figuring out how large changes in these interactions affect the ability of structures like chiral magnetic domain walls to reliably store data. “We want to identify the main interactions needed to make stable structures, which can then be used to store data in future commercial devices,” says Meijer.
But chiral magnetic domain walls aren’t the only magnetic racetrack structures of interest to Meijer and her colleagues who are trying to figure out how to store data in these structures. There are spin spirals – periodic magnetic structures, and the coolly-named skyrmions.
Can you transform a skyrmion?
Named after Tony Skyrme, who first proposed them in the early 1960s, a skyrmion is a swirling, complex magnetic structure. Although let’s be honest – it sounds more like a character from a superhero film!
Uncontrolled transformations of skyrmions are rare since they are topologically stable. “Think of a knot in a very long rope. It would take you quite a long time to unwind or remove the knot. You can say that the knot is topologically stable,” explains Meijer. “For skyrmions, the ‘knot’ represents the data, and being topological stability means that only a very large increase in the external magnetic field or temperature could ‘unwind the knot’ and delete the data.”
This topological stability makes skyrmions, as well as chiral magnetic domain walls and spin spirals, ideal for data storage in magnetic racetrack memory. These structures rotate, and how they rotate is affected by their local environment and influences the data stored in the structures. But it’s only recently become clear that measuring rotations is important.

Whirling imprints
It was long assumed that magnetic structures in between domains (the so-called domain walls) all rotated in the same way, but this is incorrect. As such, accurate measurement of the swirling of these magnetic structures is essential.
“To measure the rotation of the whirling structures in the lab, we need a technique that has a resolution of 10 to 20 nanometers – the typical size of domain walls. That’s why we use scanning electron microscopy with polarization analysis (SEMPA),” explains Meijer.
In SEMPA, the magnetic materials are scanned with an electron beam, leading to the emission of secondary electrons from the magnetic materials. The rotation of the magnetic domain walls is imprinted on these secondary electrons. Rotation information on the whirling of magnetic structures is then extracted from the electrons.
Experimenting on a Friday afternoon
Meijer and her colleagues have made some big discoveries on the interactions between rotating magnetic structures, and these findings have been published in prestigious journals such as Physics Review Letters (PRL) and ACS Nano Letters.
But it’s the paper in ACS Nano Letters that has a great backstory. Meijer: “It was a ‘Friday afternoon experiment’, just an idea, a real shot in the dark. Marcos Guimarães (former postdoctoral researcher in the same group as Meijer) and I decided to try something out and it worked! Honestly, we were both quite surprised.”
In their ‘Friday afternoon experiment’, the pair used scotch tape to strip 2D layers from the magnetic material Fe3GeTe2 – a material that was only discovered a few years ago. They then imaged how spin spirals changed in the 2D layers using SEMPA. Information on the interactions between neighbouring spin spirals could prove useful in the development of future ultrathin computing devices.
Getting to the final lap
The final lap for magnetic racetrack memory is a commercial device that anyone, anywhere can use to access data in an efficient and super-fast manner.
However, Meijer says this will take time: “We still don’t fully understand how the various interactions affect the tiny data-storing magnetic structures on racetrack memory. At TU/e, we’ve made important progress, but there’s so much more to figure out. It might be years before magnetic racetrack memory becomes commonplace in our devices.”
Before we have magnetic racetrack memory that can read and write data in less than 10 nanoseconds, patience is of the essence.
The race for magnetic racetrack memory is not over yet.