New numerical method simulates properties of scratches on wafers
Sven Sperling defended his PhD thesis at the Department of Mechanical Engineering on April 18th.
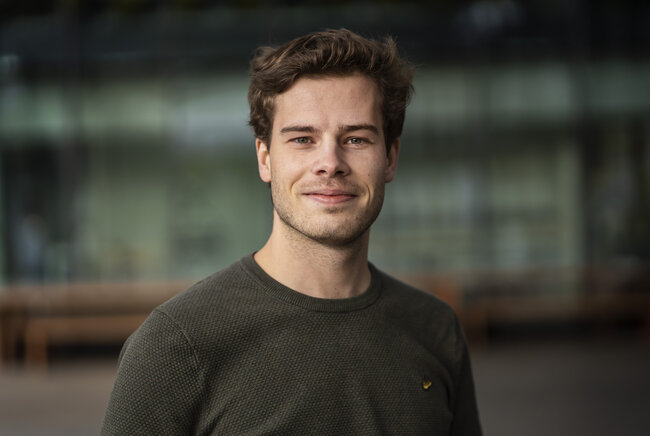
In his PhD research Sven Sperling established a microscopic scratch model for silicon. For this a material model has been extended to include the complex material behavior of silicon under contact conditions. This new numerical method can simulate the properties of scratches. It has been shown that the model in its current form simulates experimental observations well within a normal force domain of 20-50 mN. By now two papers have been published on the model in leading journals for (numerical) mechanics. This project is mainly related to further developments in the semiconductor industry, specifically for the machines made by ASML. The ACCESS consortium is the first collaboration between TU/e and VDL ETG. This research field is a new territory for both VDL ETG and TU/e.
The most popular material for the production of high-end electronic devices, such as chips and solar panels, is silicon because of its semiconducting properties and vast abundance. For the fabrication of chips and solar panels, thin slices of crystalline silicon are employed, which are called wafers. In order to convert silicon wafers into reliable products, the surface characteristics of these wafers need to be formidable, which is why wafer fabrication methods such as sawing and griding are thoroughly investigated nowadays. Also, in recent years, the subtle contact between wafers and machine components for handling has become a topic of interest since it can introduce contaminating wear debris which may interfere with the lithography process. Consequently, the reliability of the chips decreases due to manufacturing errors.
Role of numerical simulations
To investigate the implications of contact between a saw, griding device or machine component with the wafer, scratch experiments are performed on silicon substrates. From the post-scratch analysis, the surface damage can be investigated providing information on, for instance, the scratch conditions that cause surface crack formation or wear particles. However, what happens beneath the surface during scratching is unclear and therefore the mechanisms behind crack and wear particle formation remain unknown. Since experimental procedures are limited in the ability to investigate the subsurface deformation evolution during scratching, numerical simulations can play an instrumental role. But, to arrive at a reliable scratch model, three aspects need to be considered. First, a suitable numerical methodology that can account for the features that belong to scratching such as complex fracture and large local deformations. Secondly, a representative material description that can account for the mechanics of crystalline silicon under contact loading conditions at the microscopic scale. Lastly, experimental data to perform a parameter identification and validation comparison with the simulations. These three subjects are covered in detail in this thesis.
Dominant continuum behavior
The continuum-particle methodology adopted in this thesis allows to implement a microscale material formulation such that the computational cost maintains within a reasonable timeframe. All the while, the discrete nature of the numerical method allows to extend the model to incorporate complex fracture events by simply eliminating the bonds between particles. The focus in this thesis, though, is to capture the scratch regime in which the continuum behavior is still dominant, with the possibility to extend to larger scratch regimes including fracture and wear particle detachment.
At the microscopic scale, under compressive contact conditions, silicon exhibits a series phase transformations which are accompanied by large volumetric changes. The microscopic continuum description of the material behavior is formulated such that it includes this important but complex physical phenomenon. The microscale continuum material description is implemented in the continuum-particle methodology to perform scratch simulations, where the simulations are compared to scratch experiments which are executed under similar loading conditions. It was shown that the post-scratch surface profiles of the simulations and the experiments for a blunt indenter tip at a normal load range of 20-50 mN were in adequate agreement with each other. Also, it was shown that the phase transformation behavior which was included in the microscale material description has a significant influence on the resulting post-scratch topology. With this confirmation, further investigation into subsurface stresses and deformation behavior of silicon micro-scratching is possible.
Research School: EM (Engineering Mechanics)
Consortium: ACCESS (a collaboration with VDL ETG, together with 2 other PhD projects)
Title of PhD thesis: A continuum-particle simulation framework for silicon scratching at the microscale. Supervisors: Prof. Marc Geers, and Dr. Johan Hoefnagels.