Formation and optimization of halide perovskite interfaces from first principles
Sofia Apergi defended her PhD thesis at the Department of Applied Physics and Science Education on October 4th.
![[Translate to English:] [Translate to English:]](https://assets.w3.tue.nl/w/fileadmin/_processed_/b/5/csm_Apergi_Sofia%20Banner%20image_6f65c075af.jpg)
Extreme weather events, such as floods and heat waves, that have been affecting many places around the world are a devastating consequence of climate change. Action must be taken if we want to slow down and hopefully reverse the damage and one of our greatest weapons in this fight is renewable energy. With this in mind, perovskite materials for solar panels have grown in popularity in the last decade; because of their potential to one day make solar energy even more widespread and approachable than it is today. During her PhD, Sofia Apergi, used computer models to study different surfaces and interfaces in perovskite devices with the aim of understanding how they form and eventually how they can be optimized for potential application in solar panels.
Metal halide perovskites comprise a class of materials with the chemical formula AMX3, where A is a monovalent cation like Cs+ or the organic methylammonium (CH3NH3+ or MA+) or formamidinium ((NH2)2CH+ or FA+), M is a metal, more often Pb2+, and X is a halide (usually I- or Br-).
In the early 2010s, these materials emerged as potential low-cost absorbers for photovoltaic devices, due to their impressive optoelectronic properties and ease of fabrication, among others. Since then, the field has grown rapidly, with perovskite solar cells reaching efficiencies comparable to those of silicon devices, which currently dominate the market.
Moreover, the use of perovskites has been expanded to several other fields, more notably light emission and detection. Despite the many benefits there is one major obstacle prohibiting the commercialization of perovskites – their persistent instability. As a result, significant research efforts are currently focusing on overcoming this instability.
Surfaces and interfaces
Perovskite devices usually comprise several layers, such as charge transporters and electrodes that surround the perovskite. Instability can often start at the interfaces between these layers; therefore, their optimization is one of the ways to increase the lifetime of perovskite devices.
During her PhD, Sofia Apergi, used atomistic modelling to study different surfaces and interfaces in perovskite devices with the aim of understanding how they form and eventually how they can be optimized.
First, she investigated how perovskites (do not) form on ZnO and MoO3, two of the most popular charge transport layers (CTLs) in perovskite devices. To do that, Apergi looked at how common perovskite precursor molecules interact with the surface of these oxides. Different reaction pathways were identified for each oxide, which degrade the perovskite and/or even the oxide layer itself. Such understanding of the origin of degradation can allow for the design of appropriate passivation strategies, and eventually mitigate instability. In the case of ZnO, MAX/FAX treatment before the deposition of the perovskite could do the trick, while for MoO3 the passivation of surface oxygen vacancies was found to be very beneficial.
Molecular modification
Next, Apergi explored the use of molecular modification as a passivation approach, aiming to enhance not only the stability, but also the performance of perovskite devices.
A very important aspect of these devices is the electronic level alignment of their comprising layers. These need to be properly aligned to ensure the efficient movement of charge carriers along the device and consequently its optimal performance.
Apergi explored twenty different alkali halides as potential surface modifiers for another CTL, namely nickel oxide (NiO). She found that with such modifiers, the electronic levels of NiO can be shifted within a surprisingly large range of energies, which could allow it to form efficient interfaces with many different perovskite compositions and for different applications.
Similarly, other organic modifiers, namely amines and a phosphonic acid, were found to be effective, not only in improving charge transport, but also in passivating different perovskites and CTLs.
Mirror symmetry
Finally, Apergi investigated how perovskites can be functionalized by interfacing them with a special class of molecules, which lack mirror symmetry.
Thanks to these so-called chiral molecules, chiral perovskites can differentiate between left- and right-handed circularly polarized light. This property can be very useful in fields, such as telecommunications and imaging.
Using atomistic modelling, she found a correlation between the exhibited optical properties and the perovskite structure.
This knowledge is a great first step in better understanding these novel intriguing properties and more importantly in being able to design chiral perovskites with desired properties.
Title of PhD thesis: Formation and Optimization of Halide Perovskite Interfaces from First Principles. Supervisors: Geert Brocks and Shuxia Tao.
Media contact
Latest news
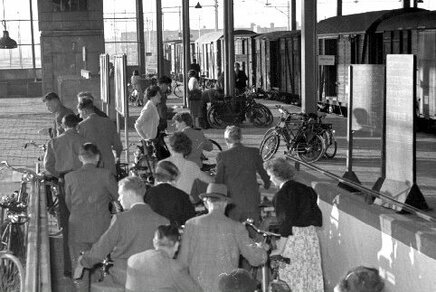
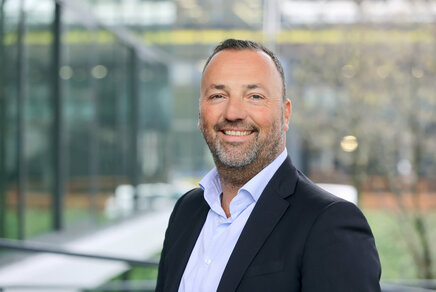
![[Translate to English:] [Translate to English:]](https://assets.w3.tue.nl/w/fileadmin/_processed_/e/0/csm_BvOF%202019_1031_BHF%20license%20TUe%20ILI%20copy_8a50884392.jpg)